The Pedestrian Dynamics research line studies the dynamics of crowds in complex urban scenarios as well as the propagation of infectious pathogens within a crowd. We work on the application and development of two independent mathematical models: a pedestrian dynamics tool based on the so-called Social Force Model (SFM) [1] to simulate the motion of agents in complex geometries and pathogen transfer models based on anisotropic diffusion equations to simulate the direct and indirect respiratory transmission. We use CFD tools based on OpenFOAM for the calculation of desired pedestrian trajectories of single agents in arbitrarily complex geometries, as well as meshless Smoothed Particle Hydrodynamics (SPH) concepts for the “on-the-fly” estimation of local pedestrian densities and the propagation of the viral load between agents.
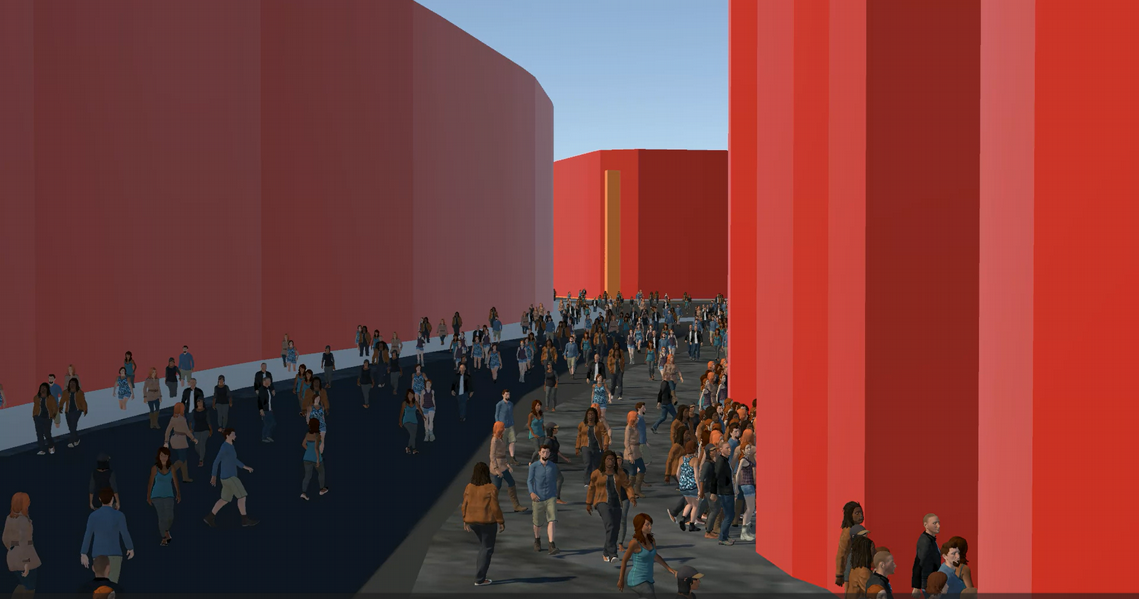
Fig 1: Snapshot of football fans entering the San Mamés stadium in Bilbao.
Anisotropic Social Forces models
Building upon existing versions of the SFM, we have studied the macroscopic and microscopic effects of contact and social repulsive forces, being able to reproduce two of the most critical sets of macroscopic empirical data in the field of pedestrian dynamics: the speed-density fundamental diagram (FD), and the flow-width relation in evacuations (BF) (Fig.2). We have shown the importance of considering the anisotropic pedestrian interactions to reproduce both the FD and BF test cases: pedestrians reaction to obstacles is gradually reduced along directions farther from their line of sight. Furthermore, the calibrated model reproduces correct collective patterns such as collision avoidance in a wide range of relative velocities, arch formation before bottlenecks, and lane formation in bidirectional crowd flows [1].
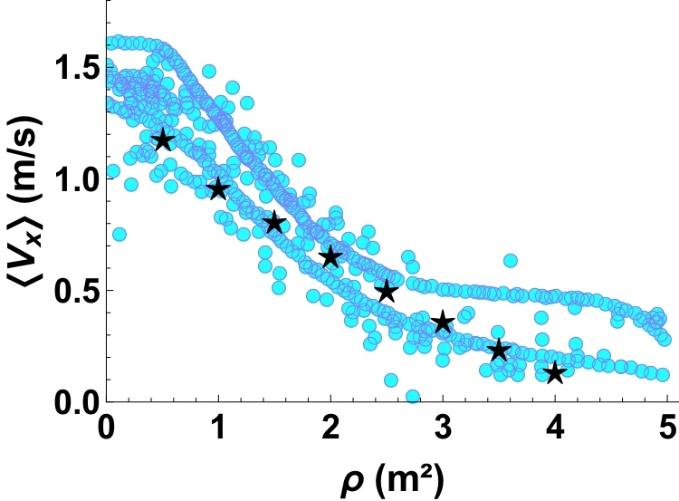
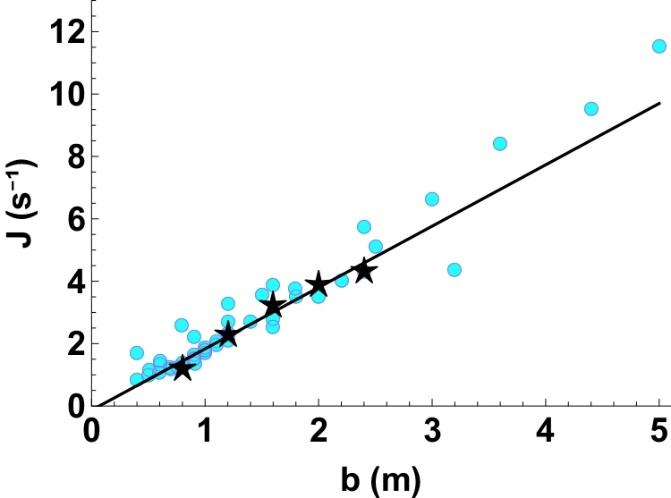
Fig. 2: Reproduction of FD (left) and BF (right) empirical data in cyan, with a single version of the Social Force Model [1].
Rheology of a crowd
More recently, we have proposed a novel approach to understand pedestrian dynamics at continuum level by taking advantage of the close analogy between pedestrian systems and (non)Brownian suspensions. We have carefully studied the Faster-Is-Slower (FIS) phenomenon, which occurs in pedestrian evacuations when individuals willing to exit faster achieve in average the opposite result, i.e. slower evacuations. As a first step, we have established a relationship between the FIS and the well-known Discontinuous Shear Thickening (DST) phenomenon in granular suspensions.
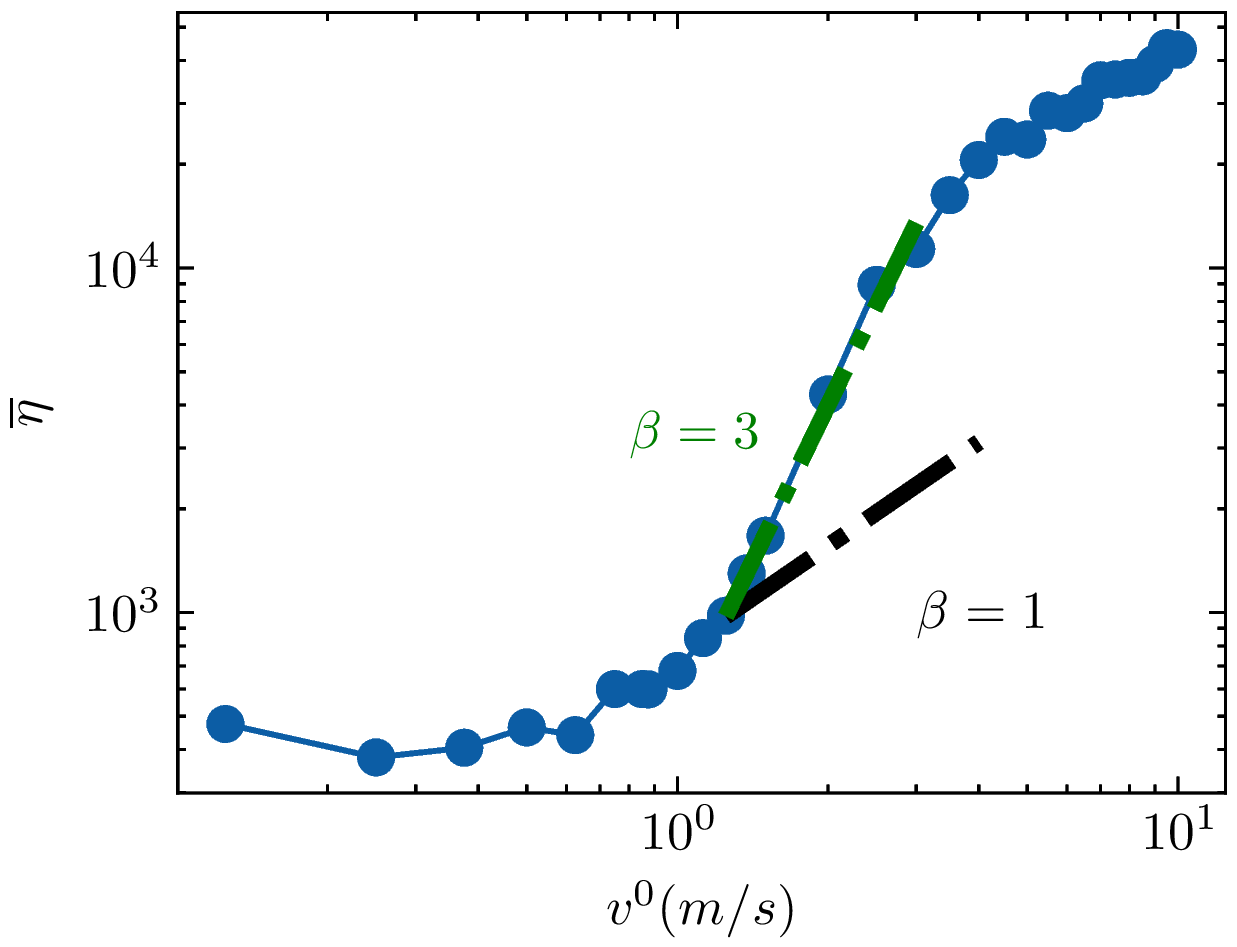
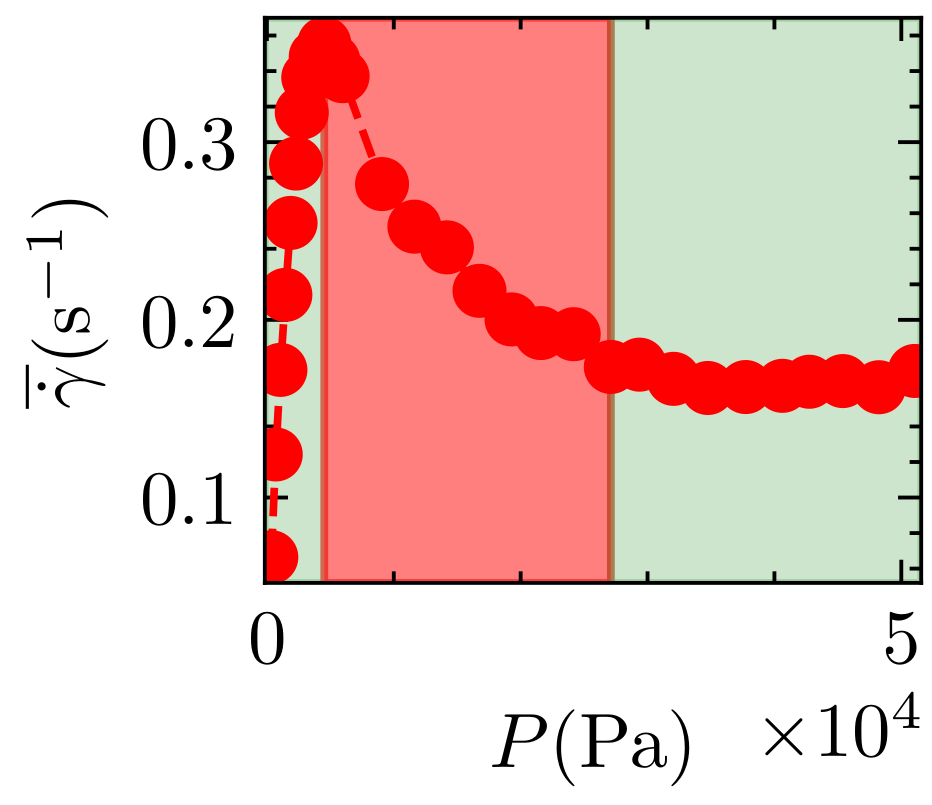
Fig. 3: Evidence of discontinuous shear-thickening (DST) rheology in crowd systems under evacuation. Left: local shear-rate against imposed crowd pressure. Right: crowd flow viscosity as a function of the competitiveness of the crowd.
By simulating a system of pedestrians existing a single-exit room, in Fig. 3 (left), it is shown how the shear rate exhibits a non-monotonic behaviour with the increase of the pressure. On Fig.3 (right) it is displayed how the effective crowd viscosity increases abruptly with an exponent close to one, that denotes DST behaviour. Both outcomes are well-known fingerprints of DST observed in (non)Brownian suspensions and therefore suggests a novel way to model dense crowd at continuum level based on microstructural models of the Wyart-Cates type [3], rather than as standard granular media (Fig. 4).
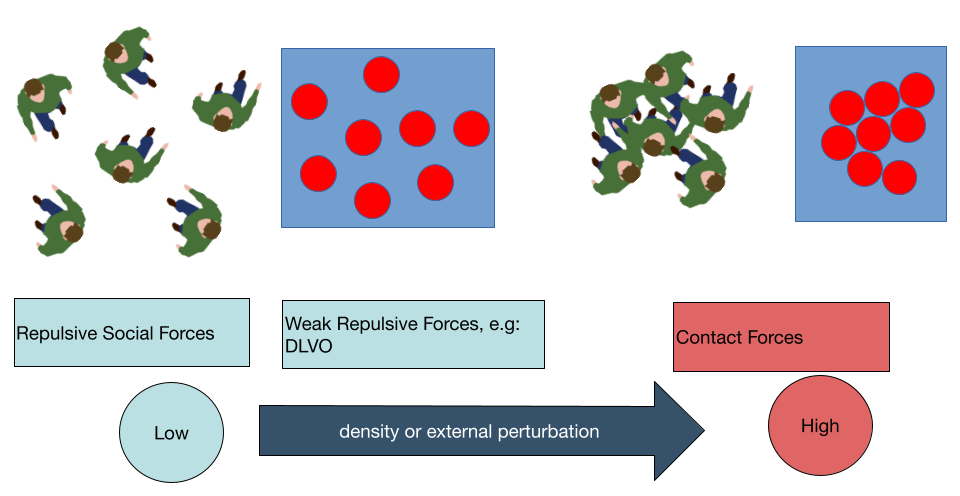
Fig. 4: Similarities between (non)Brownian suspensions and pedestrian crowds where FIS is interpreted as a frictionless-to-frictional transition mediated by a social pedestrian repulsion, in analogy with the role played by short-range repulsive forces in DST of granular suspensions [2].
Pedestrian dynamics in complex geometries
We have applied the calibrated pedestrian model to the access of football fans to a digital twin of San Mamés stadium (Fig. 5, left). In our novel framework, pedestrian trajectories are calculated using OpenFOAM: we define a velocity map for each possible goal of the pedestrian and integrate it into the simulation (Fig. 5, right)
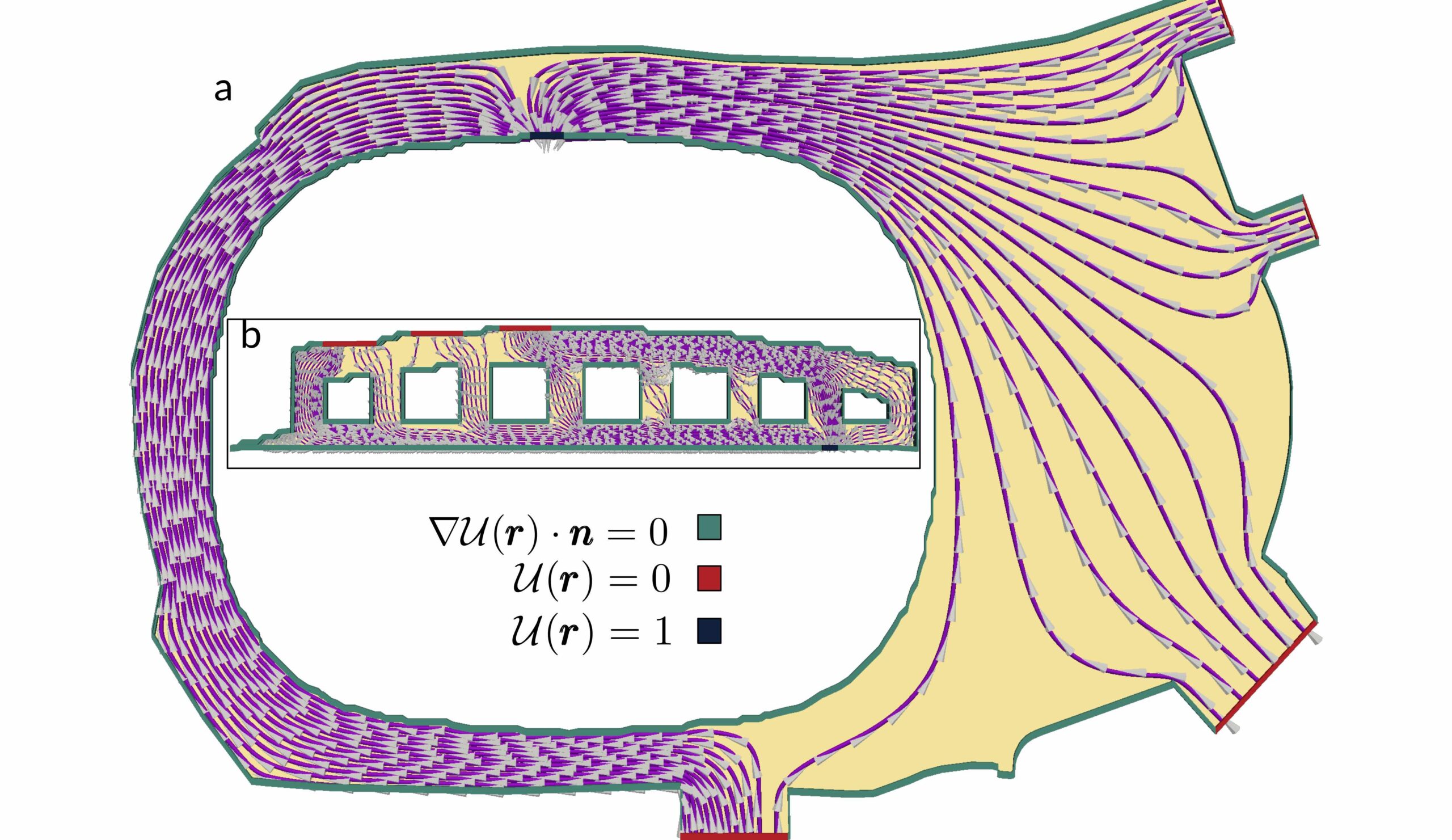
Fig. 5: Computational version of San Mamés stadium. Desired velocity field for pedestrians in the complex outdoor area of the stadium and indoor (inset) precomputed using OpenFoam CFD.
This way, our group has reproduced the access of football fans to the turnstiles of San Mamés stadium, comparing numerical results with actual data from 15 different matchdays provided by Athletic Club (Fig. 6, left). Then, we have assessed the high local densities that can be formed depending on the arrival rate of people and the total number of attendees (Fig. 6, right) [3].
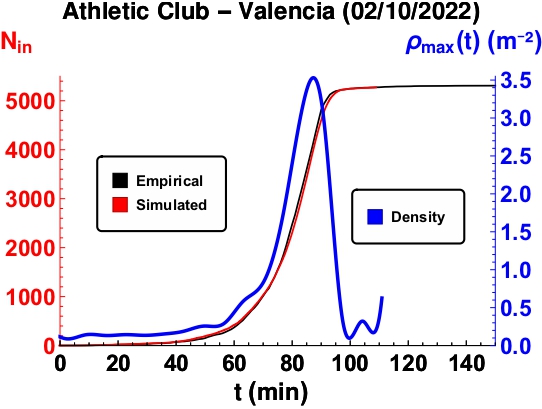
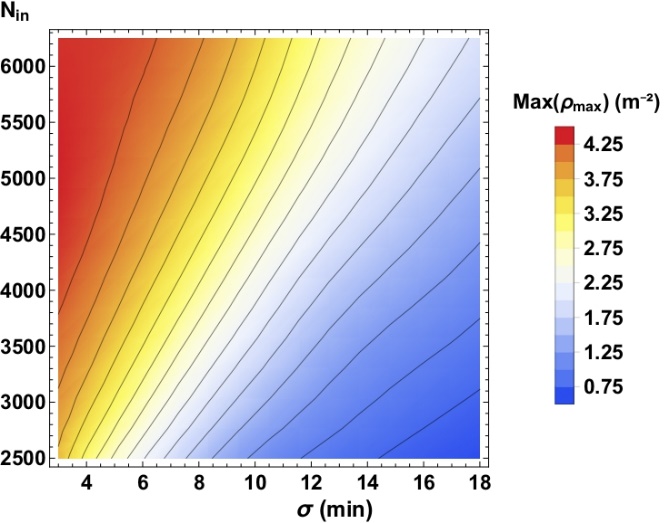
Fig. 6: Left: Empirical and numerical cumulative sum of fan access through turnstiles, and temporal evolution of maximum local pedestrian density. Right: Contour map of the maximum local pedestrian density as a function of the total number of attendees and the standard deviation of their arrival rate.
Infection Dynamics
More recently, our research has shifted towards developing a pathogen transmission model for viral diseases, including the complexfluid dynamical nature of their spread. This model can be easily coupled with the pedestrian dynamics model to simulate the contagion of a disease within a crowd moving in urban scenarios and assess to which extent mobility can reduce the transmission of the viral load.
We build upon the diffusion equation treated with smoothed-particle hydrodynamics to simulate the diffusion between pairs of agents. Three kinds of agents make up the virtual environment: healthy (susceptible agents, with viral load equal to 0, and exposed agents, with viral load between 0 and 1) and infected (viral load equal to 1). Only infected agents can transmit the disease, either directly or indirectly.
Direct transmission occurs when a susceptible or exposed person comes into the cut-off radius of the infected individual. The face-to-face orientation strongly affects the transmission: the emitter exhales pathogen in a conical jet that is very localized in the direction of his/her mouth, and the receiver inhales more pathogen the closer the jet direction is towards his/her face. This contagion mechanism is also inversely proportional to their relative distance and to the density of pedestrians around each agent, following the SPH discretization of the diffusion equation.
Indirect transmission has two steps. First, infected agents increase the viral load of the room where they are located. Then, healthy agents enter the room and inhale the pathogen that is concentrated in the environment. The room loses viral load not only when healthy agents inhale it, but also after each time step following and exponential decay of viruses proven by the literature.
The combined model is expected to be applied to the assess the effectiveness of the measures taken during the COVID-19 pandemic in the Galdakao-Usansolo Hospital in Biscay (see Fig. 7), comparing the working day motion of healthcare workers before and during the pandemic.
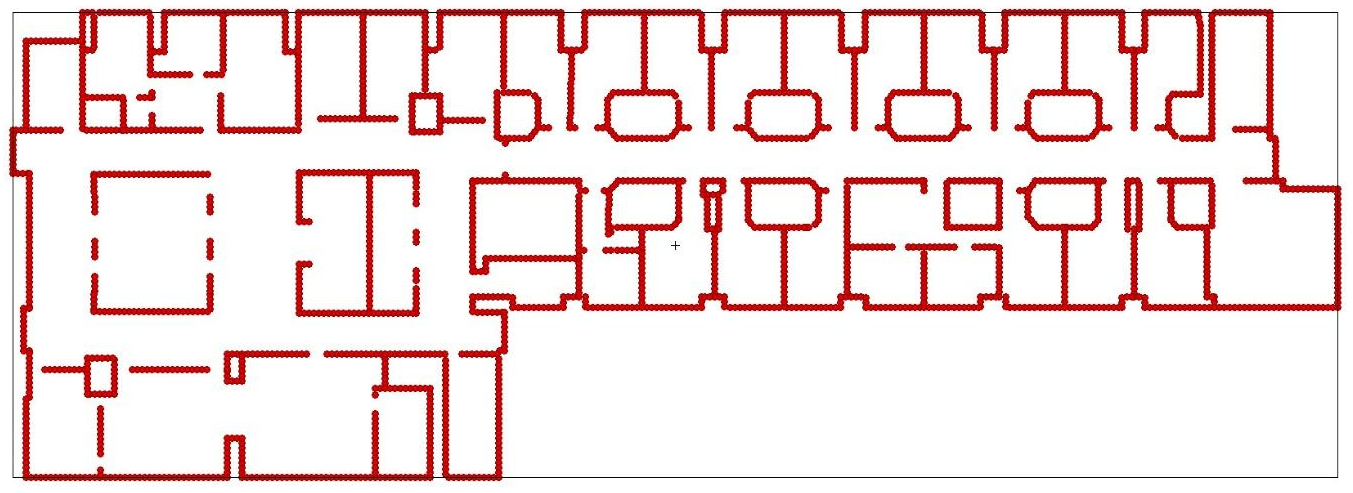
Fig. 7: Virtual geometry of the 9B floor in Galdakao-Usansolo Hospital.
Current areas of application include the definition of a platform to manage and organize massive events in the province of Bizkaia and the assessment of the transmission of COVID-19 in Galdakao-Usansolo Hospital.
References
[1] A. García, D. Hernández-Delfin, D.-J. Lee, and M. Ellero (2023). Limited Visual Range in the Social Force Model: Effects on Macroscopic and Microscopic Dynamics, Physica A: Statistical Mechanics and Its Applications 612, 128461, doi https://doi.org/10.1016/j.physa.2023.128461
[2] D. Hernández-Delfin, A. García and M. Ellero (2023). Rheology of a Crowd: from Faster-Is-Slower to Discontinuous Shear Thickening, Comm. Phys. under review.
[3] A. García, D. Hernández-Delfin, B. Gonzalez, G. Garitaonaindia, D.-J. Lee, and M. Ellero (2024). Digital Twin-Enhanced Analysis of Local Fan Density During Football Stadium Access: Integrating Pedestrian Flow Simulations and Empirical Data.
[4] A. García, D. Hernández-Delfin, C. Echeandia, J. M. Quintana, D.-J. Lee, and M. Ellero (2024). A Novel Model Based on Smoothed-Particle Hydrodynamics to Simulate the Direct and Indirect Transmission of Infectious Diseases.
[3] M. Wyart and M. E. Cates (2014). Discontinuous shear thickening without inertia in dense non-brownian suspensions. Phys. Rev. Lett., 112, 098302.