Virus Dynamics
Since virus transmissibility depends on features beyond its genetic sequence, new tools are required to discern the effects of spikes functionality, interaction, and morphology. Researchers have addressed different studies about virus genetics, biochemistry, infectivity, physics, and dynamics. This investigation focuses on the transport of SARS-CoV-2 virions using computational simulations. Due to the morphological features, virions can be seen as decorated nanoparticles and characterized according to their translational and rotational diffusivity. To this end, the Rigid Multi-Blob (RMB) method [1] and Smoothed Dissipative Particle Dynamics (SDPD) [2] were implemented to simulate the hydrodynamics effect of morphology and spike proteins on virus transport.
The previous research implemented by the group postulates the relevance of the hydrodynamic interactions in the viral infectivity of enveloped viruses and micro-rheological characterization as a platform for virus differentiation [3]. The computed diffusivity of spike-decorated structures uses a mesoscopic hydrodynamic simulation to understand how the spikes affect virion mobility and infectivity. Using the RMB conducted the simulations setting the morphologies of the virus presented in FIG 1.
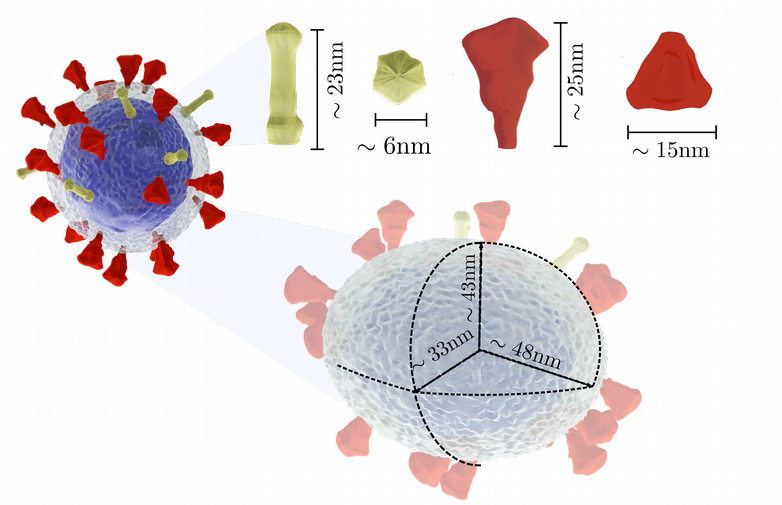
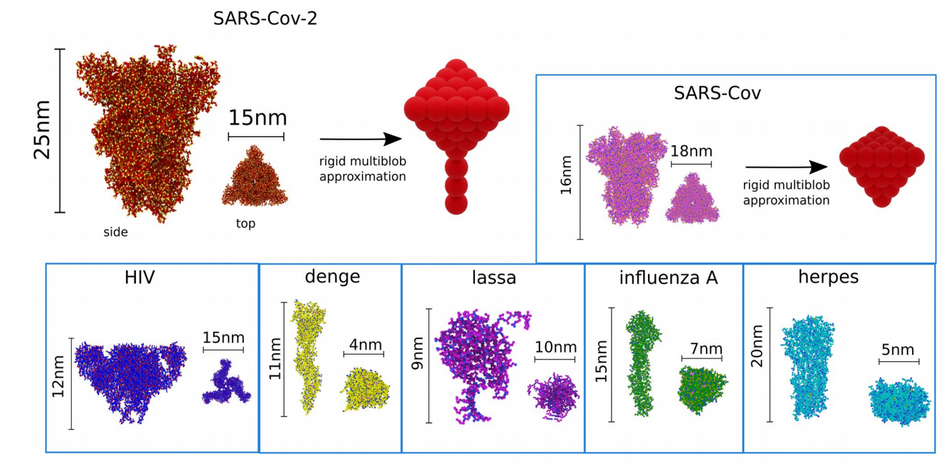
FIG 1. Discretization was adopted to model the surface-protein morphology of different virion characteristic sizes for HIV, dengue, SARS-Cov-2, SARS-Cov, Lassa, influenza, and herpes. The primary discrete morphologies are rod, tetra, sphere, rod-tetra, and rod-sphere.
Proteins’ sizes, numbers, and shapes are investigated to set these models. The computed diffusion shows that surface protein strongly affects the virion’s transport properties. Therefore, regulating the number of spikes or proteins is a possible alternative to compensate for the mobility reduction due to the spike’s size and shape [3].
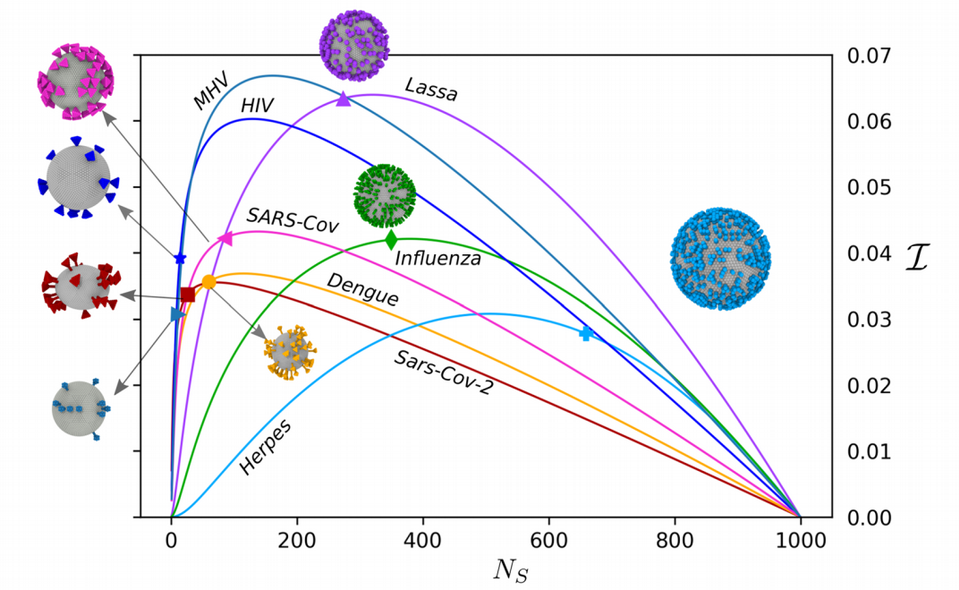
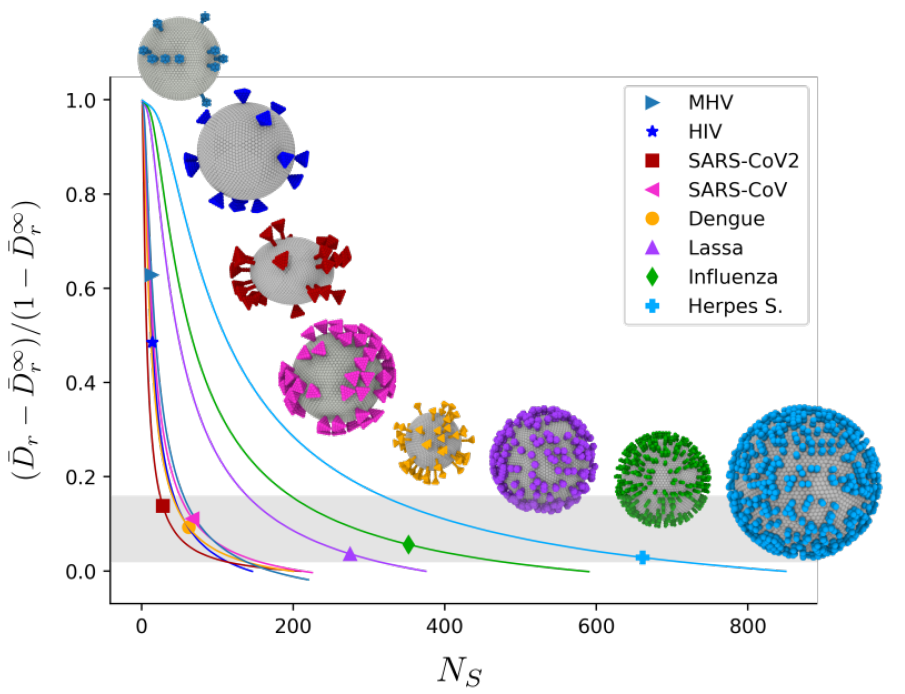
FIG 2. Variation in the infectivity parameter I with the number of spikes. Fitting curves of excess in rotational diffusivity and number of spikes.
Complex nanoparticles
Functionalized nanoparticles (NPs) are complex objects present in a variety of systems including synthetic grafted nanoparticles to viruses. The morphology and number of the decorating groups can vary widely between systems. Thus, the modelling of functionalized NPs typically considers simplified spherical objects as a first-order approximation. At the nanoscale label, complex hydrodynamic interactions are expected to emerge as the morphological features of the particles change, and they can be further amplified when the NPs are confined or near walls [4]. See FIG 3.
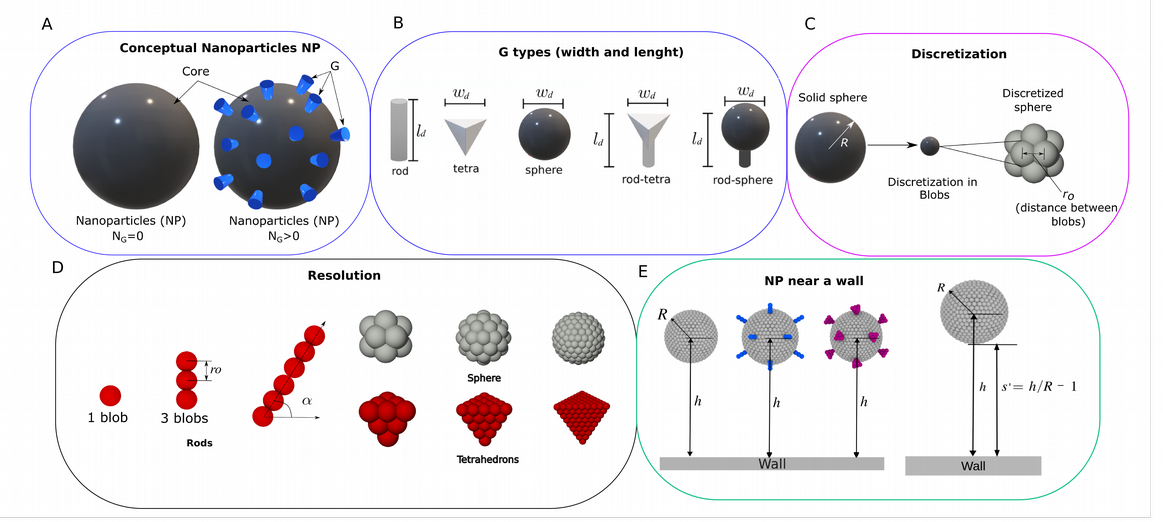
FIG 3. Sketch of a NP and the discretization adopted. A. NP representation with core and functional groups, G as blue cylinders. If the number of groups (NG) is NG = 0 the NP is only the core. B. Functionalized shapes or G types representation, the measurement of length lG and width wG are reduced by the radius of the core. C. Discretization of a sphere in blobs. D. Resolution and refinement following the G types rod, tetrahedron, and the core sphere. E. NP near a wall, the distance h is given by the wall to the center of the NP.
Direct estimation of these variations can be inferred via diffusion coefficients of the NPs. However, the evaluation of the coefficients requires an improved representation of the NPs morphology to reproduce important features hidden by simplified spherical models. Here, we characterize the passive transport of free and confined functionalized nanoparticles using the Rigid Multi-Blob (RMB) method [1],[5]. We identify that the presence of functional groups significantly affects the rotational diffusion of the nanoparticles, moreover, the morphology of the groups and number induce characteristic mobility reduction compared to non-functionalized nanoparticles [6]
Stick Slip particles
The partial-slip (a.k.a stick-slip) conditions are given by the slip length ranging from stick (no-slip) to slip (free-slip). Slip-stick particles of interest. Are setting different structures of these particles such as the classical Janus (50% slip, 50% stick), partial slip (variable average location of slip) and stripes.
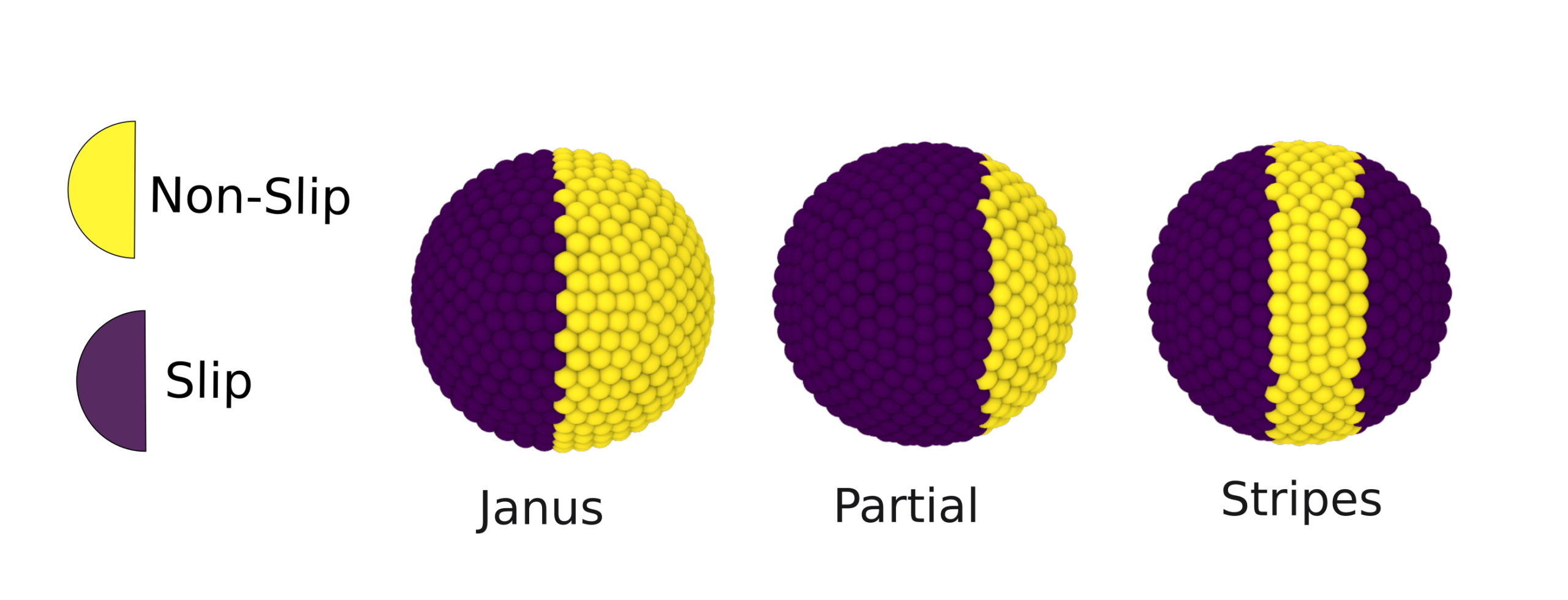
FIG 4. Stick-slip nanoparticles with structures janus, partial and stripes
The main interest are in studying the hydrodynamic interactions by variable slip-stick particles moving through a viscous fluid under unsteady conditions. To accomplish this, is implemented the computational Rigid Multi-Blob (RMB) method to simulate and model the behavior of stick-slip particles with different patterns (Janus (50% slip, 50% stick), patches, stripes). Specifically focused on understanding how a combination of no-slip and slip effects influences the characteristics of mobility, velocity, and force.
References
[1] F. Balboa Usabiaga, B. Kallemov, B. Delmotte, A. Bhalla, B. Griffith, and A. Donev, Hydrodynamics of suspensions of passive and active rigid particles: a rigid multiblob approach. Communications in Applied Mathematics and Computational Science, 2017, 11(2), 217-296.
[2] Espanol, P., & Revenga, M. (2003). Smoothed dissipative particle dynamics. Physical Review E, 67(2), 026705.
[3] Moreno N, Moreno D, Balboa Usabiaga F, Ellero M. Hydrodynamics of spike proteins dictate a transport-affinity competition for SARS-CoV-2 and other enveloped viruses. doi: https://doi.org/10.1101/2022.01.03.474721
[4] X. Bian, S. Litvinov, R. Qian, M. Ellero, and N. Adams, Physics of Fluids 24 (2012).
[5] B. Sprinkle, F. B. Usabiaga, N. A. Patankar, and A. Donev, Journal of Chemical Physics 147, 1–45 (2017)
[6] D Moreno, N Moreno, F Balboa & M Ellero. 10.48550/arXiv.2211.16181. (2022).